MOSAiC papers pour in, revealing new findings about the data-sparse and vulnerable region
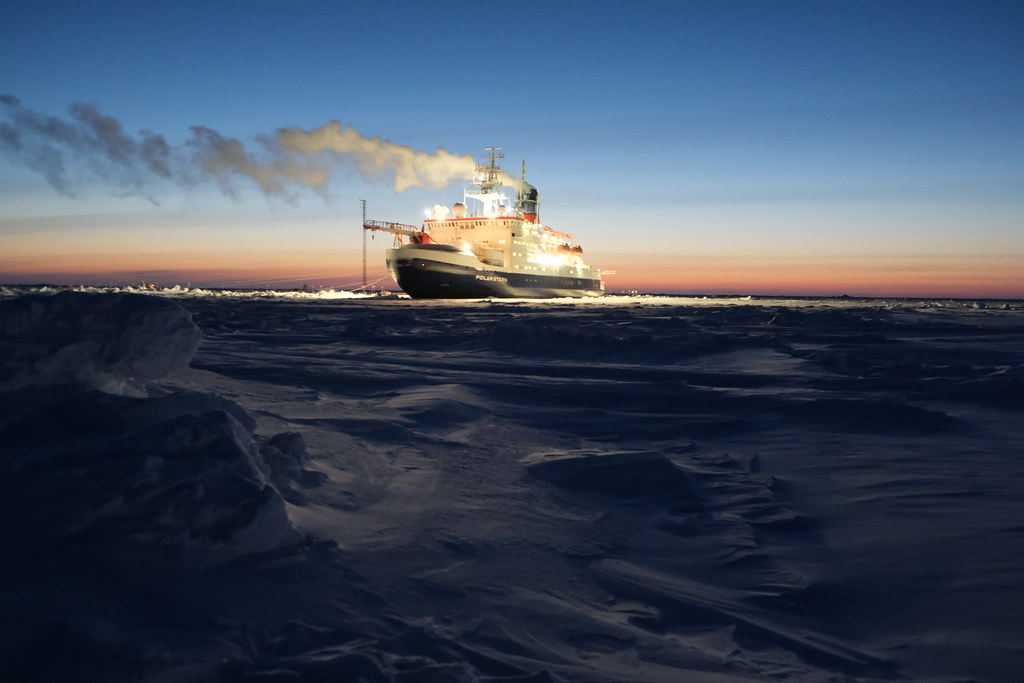
Five years ago, on September 20, 2019, the German icebreaker R/V Polarstern eased away from a dock in Tromsø, Norway, headed for its rendezvous with an ice floe. This marked the beginning of the epic international Multidisciplinary Drifting Observatory for the Study of Arctic Climate (MOSAiC) expedition, led by Germany’s Alfred Wegener Institute. The 13-month, 20-nation investigation went on to amass terabytes of rare data on the Arctic’s atmosphere, sea ice, snow, ocean, and ecosystem.
MOSAiC, served by 300 scientists working in months-long rotations, was designed to help researchers observe, understand, and ultimately model conditions in the Arctic. Temperatures there are warming nearly four times faster than the global average—what scientists call “arctic amplification”—and sea ice in the region is shrinking by 13% each decade.
The U.S. Department of Energy (DOE) was a significant contributor to MOSAiC. DOE’s Atmospheric System Research (ASR) program provided key research funding.
And DOE’s Atmospheric Radiation Measurement (ARM) user facility deployed more than 50 instruments on and around the Polarstern, which was frozen into sea ice to become a drifting instrument platform, laboratory, and hotel. ARM had technicians stationed in the Arctic the whole time, including the early months of the COVID-19 pandemic, to help keep the instruments running smoothly during all five legs of the voyage.
So far, MOSAiC’s contributions to science are considerable.
By the end of September 2024, for instance, ARM’s publications database recorded 97 MOSAiC-related publications, including 77 peer-reviewed papers. Nearly one-third (24) of them were published from October 2023 through September 2024.
‘Wildly Successful’
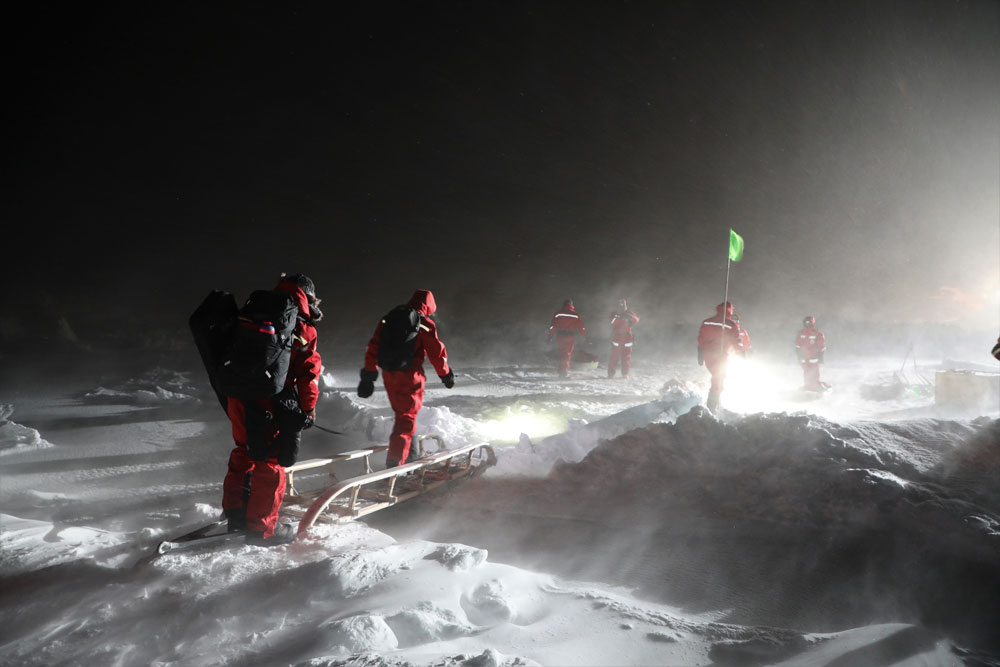
ARM data from MOSAiC, as well as all other past campaigns, are freely available to researchers worldwide in the ARM Data Center.
To date, ARM MOSAiC research has boosted scientists’ understanding of ice-nucleating particles (INPs) and ice formation in arctic clouds. Researchers also know more about arctic snowfall rates, surface energy budgets, and the annual cycle of aerosol size distribution.
ARM data have provided insights on boundary-layer stability and warm-air intrusions. They have also have pointed to new connections between sea salt aerosols and blowing snow, as reported in a 2023 paper led by Xianda Gong, now at Westlake University in China.
“Overall, MOSAiC has been wildly successful,” said the University of Colorado Boulder’s Matthew Shupe, an ASR-funded scientist who co-coordinated the expedition and led the ARM MOSAiC deployment. “DOE observations are interwoven into a lot of the research, and DOE was such an important, foundational component of the expedition.”
Shupe led a 2022 overview study of MOSAiC’s look at the atmosphere over the central Arctic Ocean. The paper has 149 citations and is designated as a Highly Cited Paper by the Web of Science research platform. To earn this designation, a paper must belong to the top 1% of papers in a research field published in a specified year.
Filling Knowledge Gaps
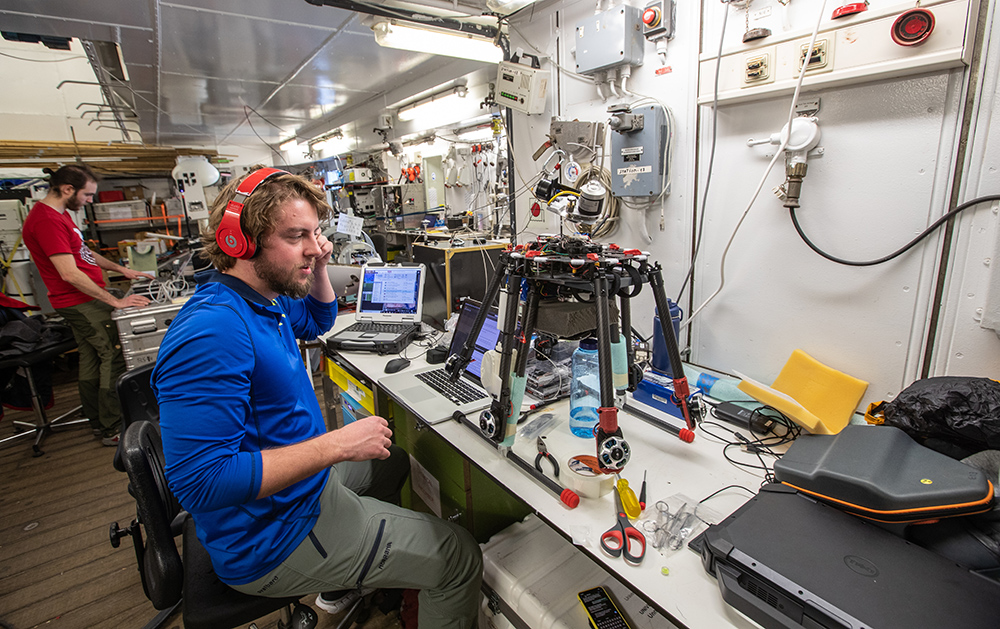
ARM data, says Shupe, have played a prominent role in filling knowledge gaps about the Arctic Ocean’s atmosphere. However, questions linger about large-scale circulation systems, aerosol-cloud interactions, heat fluxes, the relationship between sea ice and clouds, and the structure of the atmospheric boundary layer (ABL). In this turbulent region, the Earth’s surface and atmosphere exchange heat, moisture, and momentum.
In the central Arctic, it’s important to understand the ABL’s vertical structure for reasons described in a 2024 paper citing ARM data. The ABL is a critical interface between a warming atmosphere and thinning sea ice.
The paper’s lead author is Gina Jozef Hamilton, now a senior research scientist at Lockheed Martin Space.
As a pilot of uncrewed aerial systems (UAS) during MOSAiC in early 2020, Hamilton flew data-collection transects over cracks in sea ice, which expose open water and have unknown effects on the ABL.
“We collected some of the higher-resolution boundary-layer measurements that exist in the middle of the Arctic,” she says.
Hamilton’s workhorse UAS for altitudes up to 1 kilometer (just over half a mile) was the 1.6-kilogram (3.7-pound) foam-plastic DataHawk2. Its particulars are described in a 2022 paper led by another MOSAiC veteran, Jonathan Hamilton, her spouse. He is an associate scientist now on military leave from the Cooperative Institute for Research in Environmental Sciences (CIRES) at the University of Colorado Boulder.
SHEBA Parallels
“We now have two full yearlong albedo records. And it’s remarkable how similar those records are (regarding) the progression of summer albedo. We learned a lot about the mechanics of albedo evolution.”
One abiding knowledge gap in the Arctic Ocean relates to surface albedo, a measure of solar reflectivity. In summer, because of their high albedo (whiteness), sea ice and snow reflect solar radiation more efficiently than the darker open water. With a decline in snow cover and sea ice extent, arctic warming accelerates.
A 2022 paper, co-authored by Shupe and using ARM data, looked at how MOSAiC’s hourly and monthly measurements of surface albedo could reduce uncertainties related to fluxes in radiative energy—an important factor in arctic amplification.
Some of those albedo values were captured in aerial surveys by Jonathan Hamilton, who piloted a UAS hexacopter, the six-bladed HELiX, during his spring-summer 2020 tour aboard the Polarstern. The automated grid patterns the HELiX flew were often just 10 meters (33 feet) off the ground.
These flights helped provide new high-resolution perspectives on surface albedo not previously obtainable through surface- and satellite-based observing methods. HELiX flight data were featured in a 2023 study of albedo and melt-pond fraction led by MOSAiC UAS ground station operator Radiance Calmer, now at the Swiss Federal Institute of Technology.
Collecting albedo data was also a focus of the 1997–1998 Surface Heat Budget of the Arctic Ocean (SHEBA) campaign, a 20th-century counterpoint to MOSAiC.
During SHEBA, the Canadian Coast Guard icebreaker Des Groseilliers was embedded into an ice pack northeast of Prudhoe Bay, Alaska. Ringed by instrumented ice camps, the ship became a drifting observatory. ARM, then in its infancy, played a small but important part in SHEBA.
“We now have two full yearlong albedo records,” says University of Washington polar researcher Bonnie Light of the two expeditions. “And it’s remarkable how similar those records are (regarding) the progression of summer albedo. We learned a lot about the mechanics of albedo evolution.”
A Comprehensive Approach
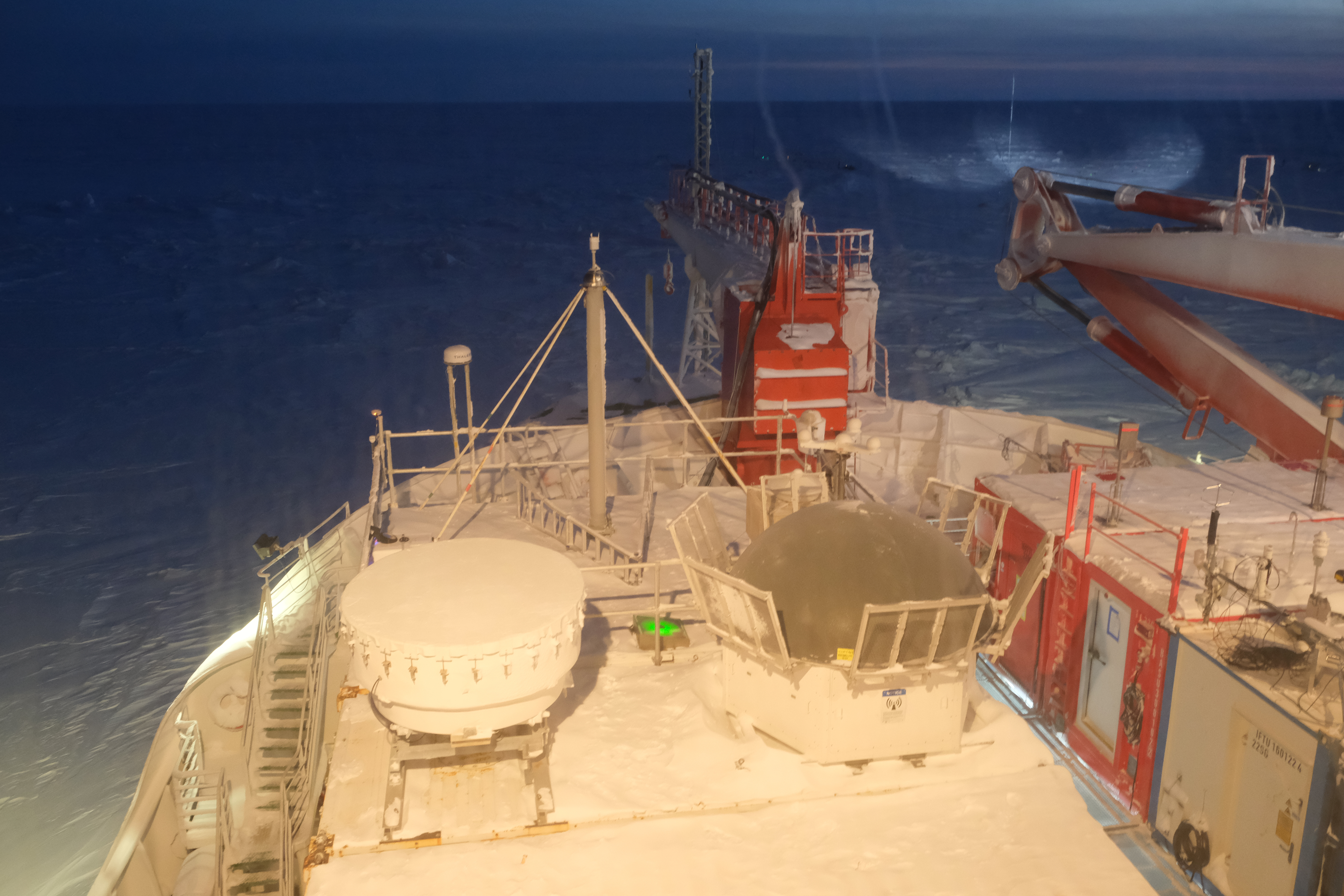
SHEBA is part of a long lineage of scientific attempts to understand the forbidding vastness of the Arctic Ocean dating to the 19th century. An 1893─1896 meteorological expedition by the oak-and-iron Norwegian ship Fram, cited by Shupe as an inspiration for MOSAiC, set the stage for quantifying changing conditions in the Arctic.
After that, a periodic Russian drifting station program (1937–2015) built a data archive on the arctic atmosphere, sea ice, and surface energy. In addition, other measurements came from a growing circle of land-based stations. Still more data came from satellite observations and occasional aircraft and seasonal campaigns. But SHEBA was first in providing rare yearlong measurements of surface energy and the cycles of cloud properties in the Arctic.
Then came MOSAiC, says Light, bringing with it improved observation technology and a comprehensive approach to collecting data.
“MOSAiC was an incredible experiment,” agrees SHEBA’s chief scientist, Donald K. Perovich, now a professor of engineering at Dartmouth College. “There has been a wealth of publications that have changed our thinking about the atmosphere-sea ice-ocean-ecological-biochemical system.”
Perovich is an expert in arctic snow cover and sea ice cover, which regulate how heat and moisture are exchanged between the Arctic Ocean and the atmosphere. He co-authored a 2024 paper that used ARM data, in part, to explore the implications for models of estimated snow thermal conductivity and conductive flux derived from MOSAiC buoy observations. Such estimates vary by space and time.
ASR co-funded the study, led by Anne Sledd, a postdoctoral researcher at CIRES.
MOSAiC and Modelers
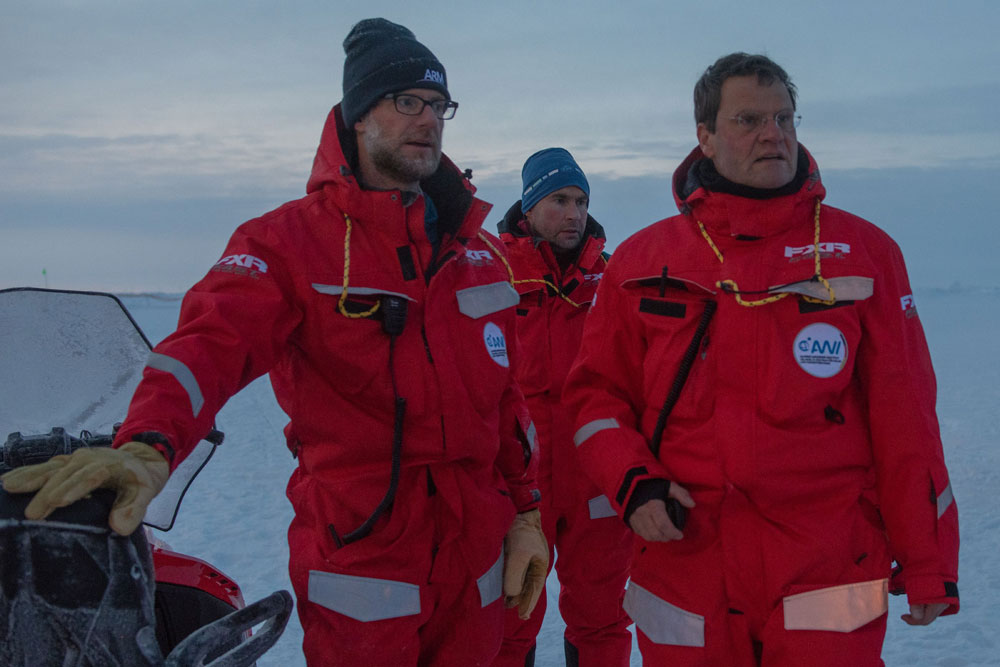
For climate modelers, the utility of MOSAiC’s post-observation analysis phase has only just begun, says Perovich. “The data are the legacy and will be used for years to come.”
A lot of expedition measurements are getting attention from modelers, agrees Shupe, including data from radiosondes launched by ARM and other entities. Modelers are using the radiosonde measurements to get baseline atmospheric profiles of air temperature, humidity, pressure, and wind patterns.
Modelers also are tapping into MOSAiC cloud data products hosted in the ARM Data Center that largely depend on measurements from instruments such as the Ka-Band ARM Zenith Radar, which operated on the Polarstern. One example is the ShupeTurner cloud microphysics product developed with DOE support. Based on observations from MOSAiC sensors, this product includes vertical views of cloud type, condensed water content, and hydrometeor particle size.
ARM MOSAiC data have been appearing in modeling-related papers.
MOSAiC’s full year of radiosonde data informed a 2022 trajectory modeling study of the life cycles and properties of stratiform liquid-bearing clouds in the Arctic. It was funded by ASR and led by Israel Silber of Pacific Northwest National Laboratory in Washington state. Silber’s paper provided critical information on short-duration cloud evolution, which is needed to simulate future climate states in a rapidly warming region.
Of the five papers Gina Jozef Hamilton led since MOSAiC ended, two touched on the needs of modelers. Both included ARM measurements in the acknowledgments.
One, in 2023, described merging disparate data sets to detail properties in the Arctic’s ABL during the MOSAiC year. One of those data sets came from what the paper called ARM’s radiation station, which included infrared radiometers and pyranometers to measure longwave and shortwave radiation, respectively. The aggregated data set is available online.
The second Hamilton paper, from 2024, relates to her PhD work: using MOSAiC observations to evaluate how well the Coupled Arctic Forecast System (CAFS) simulates the central Arctic’s ABL. CAFS is an experimental pan-Arctic short-term weather forecasting model developed at NOAA’s Physical Sciences Laboratory in Colorado. It is based in part on the DOE-sponsored Regional Arctic System Model.
“Improving models with interdisciplinary data was one of the broad goals of MOSAiC,” says Hamilton.
Arctic Aerosols
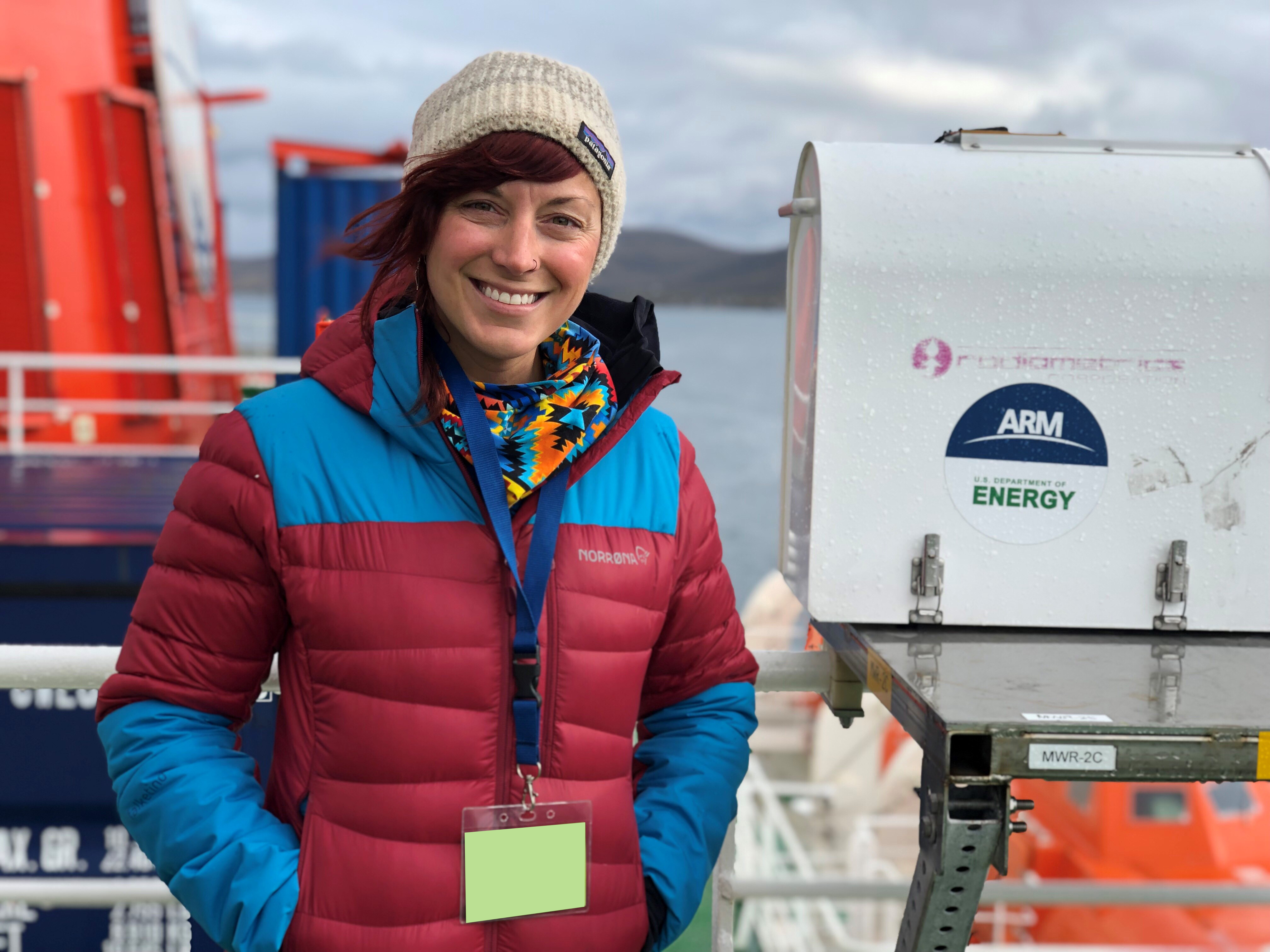
Another dimension of the expedition’s data is attractive to modelers: voluminous year-round measurements of aerosols in the central Arctic, says MOSAiC fieldwork veteran Jessie Creamean, a research scientist at Colorado State University. For ARM, she co-leads efforts to collect and analyze samples of INPs.
As part of her MOSAiC research, Creamean led a 2022 ARM- and ASR-supported study on the annual cycle of central Arctic INPs. The authors reported on the strong seasonal variability of these cloud ice precursors and how enhanced INP concentrations during the summer melt period are likely traceable to emissions from microorganisms in open patches of water.
Five years later, modelers are using the INP data to look at cloud impacts either in single cases or across the whole year, says Creamean.
A 2024 study, co-authored by Creamean, touched on biological aerosol sources such as diatoms, bacteria, and fungal spores. The impact of biota on arctic cloud formation also played a part in a 2024 paper reviewing MOSAiC ecosystem science. Creamean, Perovich, and Shupe were among the paper’s co-authors.
In related work, MOSAiC enabled a year’s worth of data on key trace and greenhouse gases, thanks in part to ARM instruments. This unprecedented trove of near-surface atmospheric chemistry measurements in the Arctic Ocean was reported in a 2022 paper.
MOSAiC in the Air
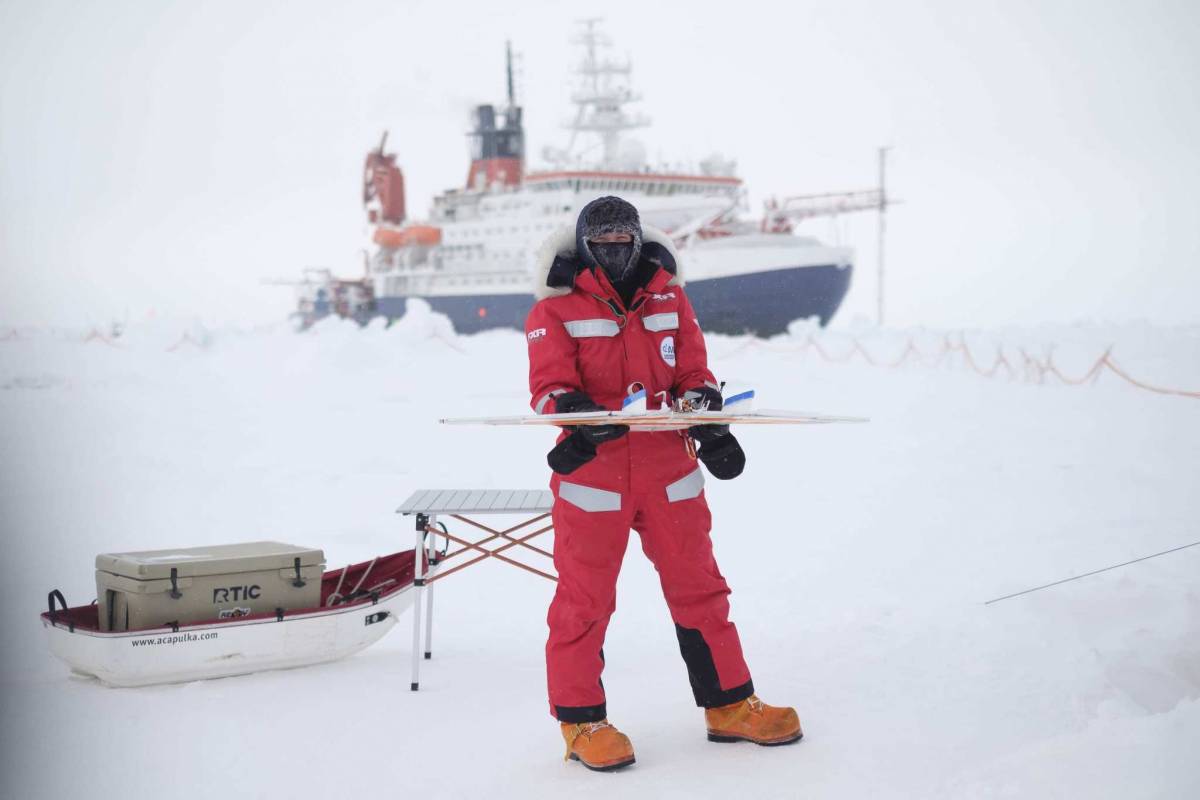
Any look back on the observation phase of MOSAiC must acknowledge the expedition’s breakthroughs in the realm of research-grade UAS. These nimble, durable flight platforms can weigh less than a pair of arctic work boots.
Gijs de Boer, a University of Colorado research scientist, oversaw the UAS teams on the ice during MOSAiC from his Colorado office. During March through July 2020, the DataHawk2 and HELiX racked up 48 hours of flight data on meteorological conditions, sea ice albedo, and melt-pond fraction.
“This was the first time UAS have been deployed in the central Arctic Ocean for such an extended period of time,” says de Boer of the half year his crews were out on the ice. “The really unique part is that we did this over multiple months at sea.”
He notes other firsts as well: the first American research UAS operating from sea ice, from a foreign vessel in the Arctic Ocean, and from a rapidly moving surface (drifting sea ice). MOSAiC also marked the first multi-month research UAS flight activity in international arctic waters, at such high latitudes, and in such cold temperatures.
After the expedition, de Boer and his UAS crews used other MOSAiC sensor systems to validate the quality of measurements from the DataHawk2 and HELiX platforms. Several of those validating sensor systems were at ARM’s radiation station, on a floe next to the expedition’s Met City research station, where ARM meteorological sensors also played a big role.
Though the UAS teams used some ARM data for validation, their research was funded primarily by the National Science Foundation and NOAA, not DOE.
It is worth noting, however, that de Boer got his first experience flying DataHawks in the Arctic through ARM-supported campaigns at Oliktok Point, Alaska. (ARM operated a mobile facility there from 2013 to 2021.) He led the Evaluation of Routine Atmospheric Sounding Measurements Using Unmanned Systems (ERASMUS) campaign from 2015 to 2016 and Profiling at Oliktok Point to Enhance Year of Polar Prediction (YOPP) Experiments (POPEYE) in 2018.
MOSAiC Echoes
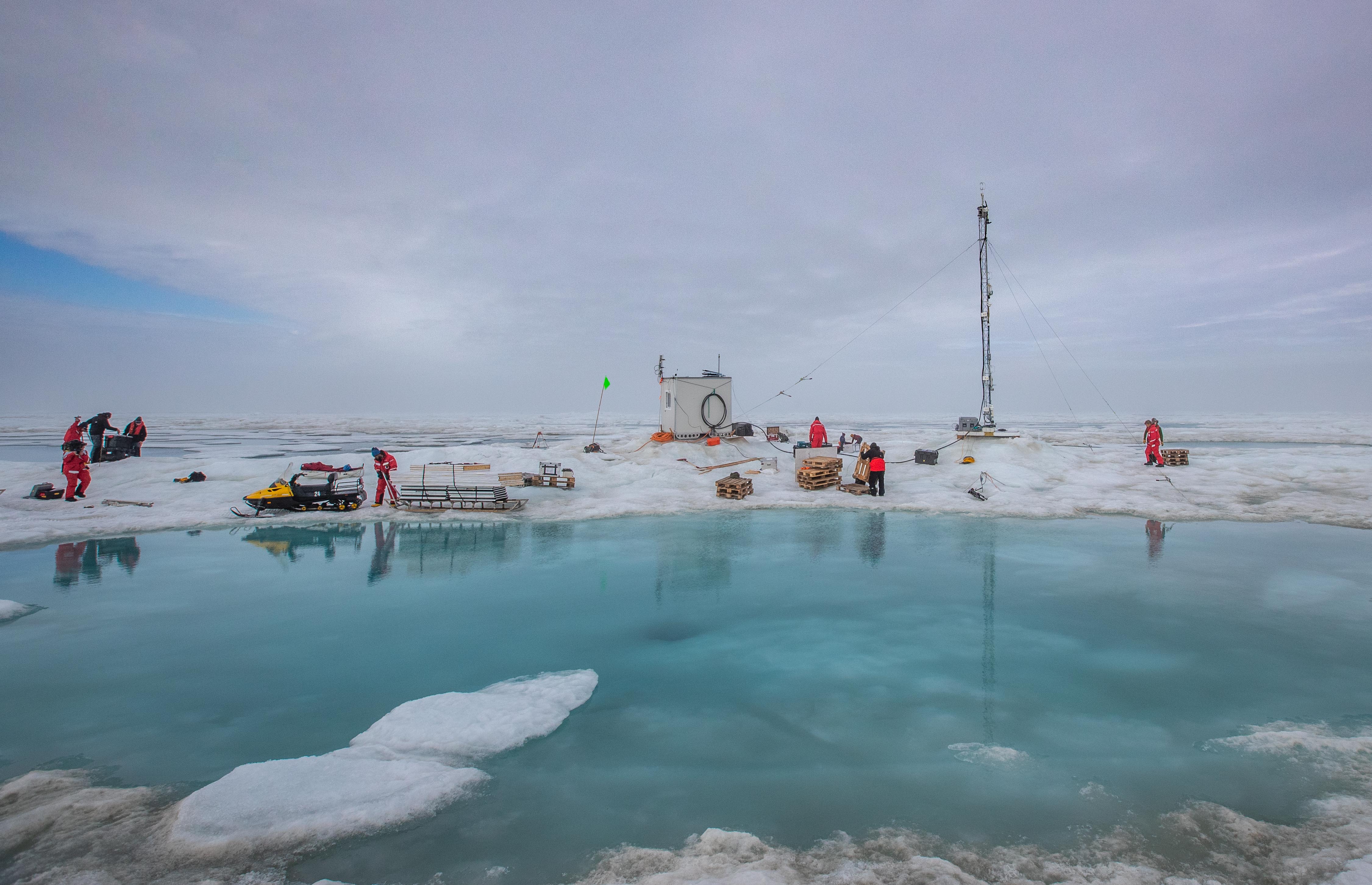
De Boer’s 2022 overview paper of the MOSAiC UAS flights laid out operational details, aircraft specifications, and data. He also described the challenges of flying in low temperatures (down to minus 35 degrees Celsius, or minus 31 Fahrenheit).
However, by the end of Jonathan Hamilton’s MOSAiC tour in July 2020, he was wading through slushy melt ponds and watching warm-air arctic fog roll in. To flee fracturing ice, UAS pilots set up their launch sites at a succession of locations.
Eventually, the atmosphere was bringing in so much heat that scientists had to abandon their on-ice workplaces. The dynamics of thinning ice and subtle wave action broke once sturdy ice floes into thousands of pieces.
The scientists’ experiences—the temperature shifts, the hazards of ice hikes, the triumph of good data—serve as a proxy for the universal MOSAiC experience, which included making solid new science friends from around the world.
Since ending in October 2020, MOSAiC has inspired fieldwork in the Norwegian Sea, Alaska, the Southern Ocean, and Antarctica. Some scientists are combining measurements from this new fieldwork with ARM MOSAiC data to better understand and model changes in the Earth’s high latitudes.
The expedition’s year of international observations meant a wide net of new work and new contacts, says Shupe. But it also meant ARM measurements getting broader attention globally.
These data, he says, “are now being used by many international scientists—in interdisciplinary research that expands beyond (ARM’s) typical domain of influence.”
# # #Author: Corydon Ireland, Staff Writer, Pacific Northwest National Laboratory
ARM is a DOE Office of Science user facility operated by nine DOE national laboratories.